A negative index material (NIM), which possesses simultaneously a negative permeability (m) and a negative permittivity (e), is an emerging material that has caught the attention of many scientists and engineers after it was first demonstrated in Science 292, 77 (2001). Many of the unique features associated with NIMs were summarized in Veselago’s original paper published in Sov. Phys. Usp. 10, 509 (1968), including negative phase velocity, reversed Doppler effect, and the prediction of a planar lens. The lack of simultaneous occurrence of negative e and m in natural materials hindered any further study on negative index materials (NIMs) for some 30 years. Micro/nanotechnology enabled the fabrication of structures with effective negative e and m in the same region. Recent studies have shown that NIMs have other remarkable properties such as the amplification of evanescent waves and hold enormous promise for applications such as the perfect lens and optical communications.
We have studied the unique radiative properties of NIMs. Photon tunneling, which relies on evanescent waves to transfer radiation energy, has important applications for radiative transfer in thin-film structures, microscale thermophotovoltaic devices, and scanning thermal microscopy [1]. By introducing NIM layers into multilayer thin-film structures, we show that photon tunneling can be greatly enhanced, due to the excitation of surface or bulk polaritons [2-4]. Energy transmission by photon tunneling between two semi-infinite dielectrics is studied using the structure shown in Fig. 1 (left). A NIM layer is assumed to insert into the vacuum gap that separates the two dielectrics. For the purpose of convenience, the modified matrix formulation is employed to calculate the transmittance of the multilayer structure. Different arrangements and numbers of NIM layers have been investigated. When an ideal NIM layer of e=m=-1 is employed, the transmittance of this layered structure is plotted in Fig. 1 (right). The transmittance becomes unity at d3=d2 regardless of the angle of incidence, wavelength, and polarization. The hemispherical transmittance is also unity since both the propagating waves and evanescent waves will transmit. Therefore, the NIM layer can significantly enhance the transmittance.

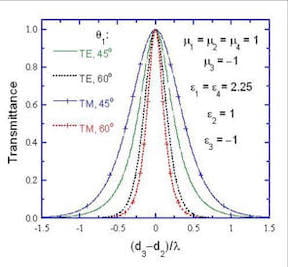
Figure 1. Transmittance enhancement by photon tunneling with a NIM [2].
The group velocity upon reflection by a NIM has been one of the controversies in understanding the optical properties of NIMs. We have investigated the direction of group velocity upon reflection by a dispersive medium regardless of whether it is a NIM or not. It was found that the group velocity is not always perpendicular to the group front [5]. We have also investigated the impact of surface and bulk polaritons on the optical properties, especially the reflectance, of a negative index metamaterial (NIM) sandwiched between different dielectric layers. Regime maps are developed to describe the polariton dispersion relations and to help understand the effect of the NIM layer thickness on the polariton resonance frequencies [6]. The reflectance from a dielectric material to another becomes zero at the Brewster angle for p-polarization. The reflectance from NIM can be zero for any polarization, that is, the Brewster angle may occur for either polarization. The criteria for the Brewster angle are determined analytically and presented in a regime map. The underlying mechanisms are explained based on the framework of the Ewald-Oseen extinction theorem [7].
We further propose a new kind of coherent thermal emission source that pairs a negative permittivity (but positive permeability) layer with a negative permeability (but positive permittivity) layer without any gratings. Coherent thermal emission can occur not only for p polarization but also for s polarization, see Fig. 2 below. Moreover, the emission frequency and emission angle can be controlled by adjusting the film thicknesses [8,9]. The findings on the spectacular radiative properties of NIMs may help develop advanced energy conversion devices.


Figure 2. Coherent emission of the proposed structure [8.9].
There exist promising opportunities for the application of NIMs to energy conversion devices and thermal radiation control. Experimentally verification of the predicted unique radiative properties is very important. Challenges exist in realizing NIMs or single negative materials in the near-infrared and visible regions.
This research has been supported by the National Science Foundation.
Publications
1. Zhang, Z.M., Fu, C.J., and Zhu, Q.Z., 2003, “Optical and Thermal Radiative Properties of Semiconductors Related to Micro/Nanotechnology,” Advances in Heat Transfer, 37, pp. 179-296.
2. Zhang, Z.M., and Fu, C.J., 2002, “Unusual Photon Tunneling in the Presence of a Layer with a Negative Refractive Index,” Applied Physics Letters, 80, pp.1097-1099.
3. Fu, C.J., and Zhang, Z.M., 2003, “Transmission Enhancement Using a Negative-Refraction Layer,” Microscale Thermophysical Engineering, 7, pp. 221-234.
4. Fu, C.J., Zhang, Z.M., and Tanner, D.B., 2005, “Energy Transmission by Photon Tunneling in Multilayer Structures including Negative Index Materials,” Journal of Heat Transfer (in press).
5. Zhang, Z.M., and Park, K., 2004, “Group Front and Group Velocity in a Dispersive Medium upon Refraction from a Nondispersive Medium,” Journal of Heat Transfer, 126, pp. 244-249.
6. Park, K., Lee, B.J., Fu, C.J., and Zhang, Z.M., 2005, “Study of the Surface and Bulk Polaritons with a Negative Index Metamaterial,” Journal of the Optical Society of America B, 22, pp. 1016-1023.
7. Fu, C.J., Zhang, Z.M., and First, P.N., 2005, “The Brewster Angle with a Negative Index Material,” Applied Optics, 44, pp. 3716-3724.
8. Fu, C.J., Zhang, Z.M., and Tanner, D.B., 2005, “Planar Heterogeneous Structures for Coherent Emission of Radiation,” Optics Letters, 30, pp. 1873-1875.
9. Fu, C.J., and Zhang, Z.M., 2005, “Further Investigation of Coherent Thermal Emission from Single Negative Materials,” submitted to Microscale Thermophysical Engineering.